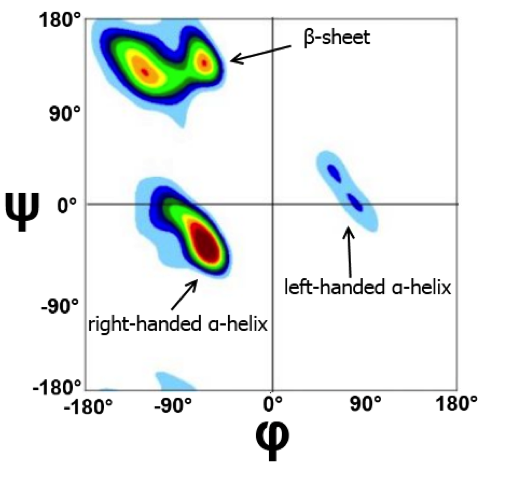
Amino Acid Structure and Shape
Notes from the edx/harvardX biochemistry class (2015)
The amino acid sequence is the protein’s primary structure. The chemical nature of the polypeptide and the constraints creates its 3D structure known as the secondary structure, which consists of β-sheets, α-helix and loops.
The polymers that make up a protein arise from condensation reactions catalyzed by the translation machinery in the cell. This leads to an unbranched polymer of amino acids residue linked by amide bonds called peptide bonds.
The geometry of peptide bonds
Looking at the peptide bond geometry, the α-Carbon (Cα) has the expected tetrahedral geometry, but the carbonyl carbon has a trigonal geometry, suggesting that the peptide bond is a double bond. Yet, its bond length of 1.32 Å, which is shorter than a single bond (1.45 Å) and longer than a double bond (1.27 Å). This is characteristic of a resonance structure.
This means that the peptide bond is planar and rigid. Meaning, it cannot rotate freely like single bonds. Because of this lack of rotational freedom, the peptide bond can only be in one of two conformations, cis and trans.
In the trans configuration, the two α-carbon atoms are on opposite sides of the peptide bond. When it’s in cis, the two R groups clash (“steric clashes”). Thus, peptide bonds are almost always trans.
An exception is an N-terminal proline: proline is cyclic, thus steric clashes are the same in cis and trans. As such, it tends to form cis.
Dihedral angles
A measure of the rotation about a bond, usually taken to lie between -180° and +180°. Dihedral angles are sometimes called torsion angles.
Φ (phi), the rotational angle between the Nitrogen and the α-Carbon
Ψ (psi), the rotational angle between the α-Carbon and the C=0
ω (omega), the angle of the peptide bond itself. Either 0 or 180 (cis, trans)
To measure the Φ look down C-N-Cα-C. To measure the Ψ look down N- Cα-C-N. Each residue has two such dihedral angles.
Ramachandran Plots
Looking for patterns in these two angles, G. N. Ramachandran recognized that many of these psi/phi combinations are forbidden because of steric collisions between atoms. The allowed values can be visualized on a two-dimensional plot called a Ramachandran diagram, which shows values of ψ and φ angles plotted against each other. Three quarters of the possible combinations are excluded by steric clashes.
Note: that the Ramachandran plots are often of sections/subsets of a protein.
So what are these things? The first of the secondary structures is the α-helix. Helices look like ribbons. In terms of Ramachandran, they appear in quadrants 2 and 3.
Each “turn” contains 3.6 residues stabilized by hydrogen bonds between the carbonyl of residue N and the amino of residue N+4 (i.e., every four residues). The peptide bonds in these helices are always φ=-57 and ψ=-47 with the side chains pointing outwards and slightly downward towards the N-terminus.
Sidebar: Linus Paulingdiscovered the α-helix in 1951.
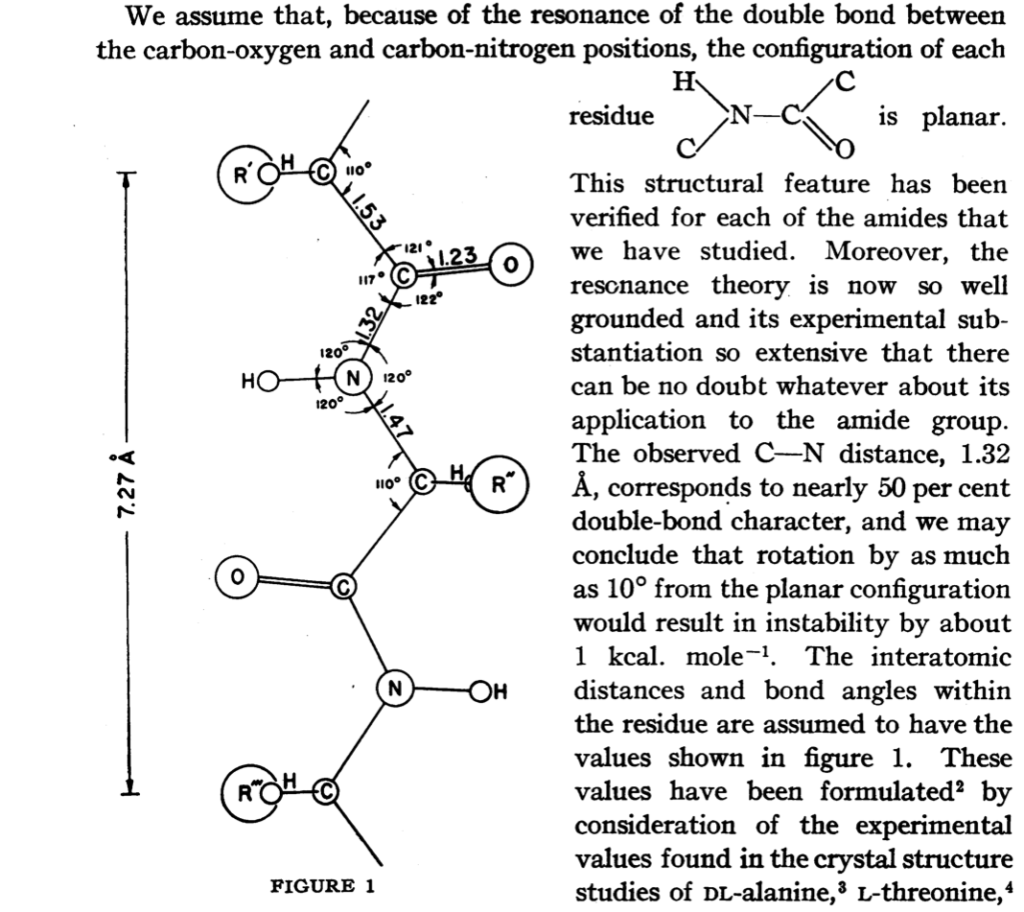
An example of an α-helix is ATP synthase, which places hydrophobic residues every 3 or 4 residues, creating a hydrophobic face to the helix. Then, by packing against a similar helix, it can form a hydrophobic core in a larger protein structure. ATP synthase will be discussed is great detail in unit three.
L-amino acids form right-handed helices. Visually, think of going up a staircase with the railing on the outside on your right. These appear in quadrant 3.
The next secondary structure is the β-sheet. A β-sheet consists of beta strands connected laterally by at least 2 or 3 backbone hydrogen bonds. These are often joined by loops at their ends.
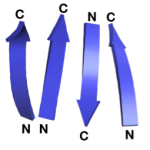
Sheets can contain parallel strands, anti-parallel strands, or mixtures. Anti-parallel strands form between adjacent parts of the primary structure joined by a short loop. They can also form from different parts of the structure or even with a different protein!
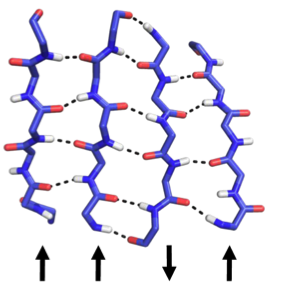
β-sheets are held together by the hydrogen bonds between adjacent strands of the backbone amino and carbonyl groups (each strand is in an extended conformation). The side chains within a strand alternate above and below the plane leading to an alternating pattern of amino acids, e.g., aliphatic and polar.
In anti-parallel sheets the hydrogen bonds are linear and the interactions between the R groups are greater that in parallel sheets. This makes them more stable than parallel sheets.
Loops can appear in all three regions of the plot. Most loops found at the surface of a protein and contain lots of polar and small molecules. They also form the shapes at active sites due to their conformational flexibility.
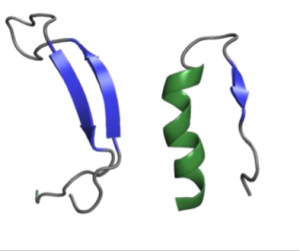
Loops often contain Gly due to its flexibility and Pro because of its unique ability to form cis bonds. They are a non-regular secondary structure.
***
Here’s an interesting aside. Can you predict the secondary structure from the amino sequence?
This is taken from Wiki:
“Amino acids vary in their ability to form the various secondary structure elements.
Proline and glycine are sometimes known as “helix breakers” because they disrupt the regularity of the α helical backbone conformation; however, both have unusual conformational abilities and are commonly found in turns.
Amino acids that prefer to adopt helical conformations in proteins include methionine, alanine, leucine, glutamate and lysine (“MALEK” in amino-acid 1-letter codes)…
….the large aromatic residues (tryptophan, tyrosine and phenylalanine) and Cβ-branched amino acids (isoleucine, valine, and threonine) prefer to adopt β-strand conformations. However, these preferences are not strong enough to produce a reliable method of predicting secondary structure from sequence alone.”
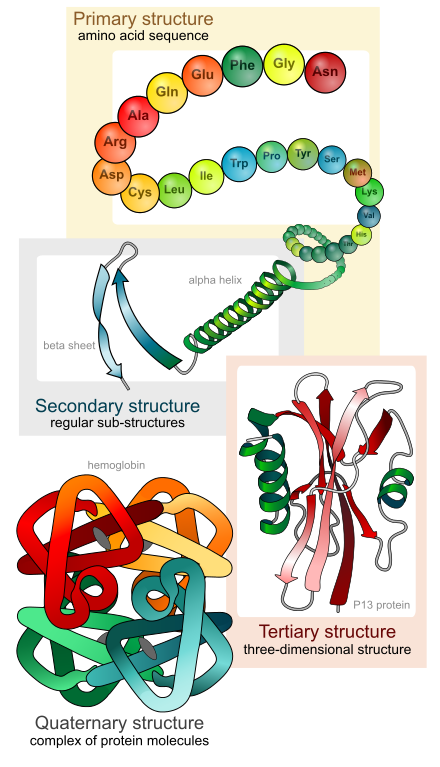
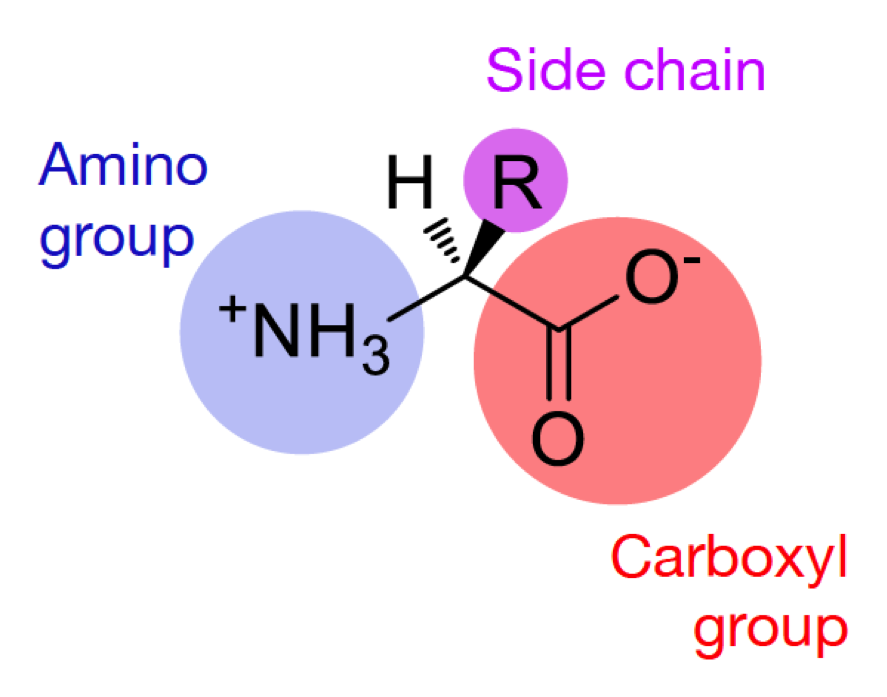